Electrolysers, Fuel Cells and Batteries: Analytical Modelling
Synopsis
Electrochemical engineering deals with electrochemical devices like electrolysers, fuel cells, and batteries. While several excellent books exist in this long-standing and still growing field, their focus is usually on chemistry or phenomenology. In this textbook, we focus on mathematical modelling of the physical phenomena involved. Instead of resorting to numerical modelling, the aim is to derive simplified analytical models that maximise understanding.
Porous electrodes, ion mass transport, and multiphase flow are central themes in this book. Examples include modelling the water saturation in a fuel cell diffusion layer, the gas fraction and current distribution in an alkaline water electrolyser, the potential distribution in a binary electrolyte inside porous battery electrode, and the concentration distribution in the flow channel of a redox flow battery. This makes for a diverse, challenging, and stimulating journey, for both students and researchers.
Downloads
References
J. B. Allen and R. F. Larry. Electrochemical methods fundamentals and applications. John Wiley & Sons, 2001
L. G. Austin. Tafel slopes for flooded diffusion electrodes.Transactions of theFaraday Society, 60:1319-1324, 1964 https://doi.org/10.1039/tf9646001319
A.J. Bard, L. R. Faulkner, and H. S. White. Electrochemical methods: fundamentals and applications. John Wiley & Sons, 2022
M.Z. Bazant. Electrochemical Energy Systems, Massachusetts Institute of Tech-nology: MIT OpenCourseWare. https://ocw.mit.edu/. License: Creative Commons BY-NC-SA., 2014. Accessed: 2021
A. Bhadra and J. W. Haverkort. The optimal electrode pore size and channel width in electrochemical flow cells. Journal of Power Sources, 579:233240, 2023 https://doi.org/10.1016/j.jpowsour.2023.233240
P. M. Biesheuvel, S. Porada, and J. E. Dykstra. The difference between faradaic and non-faradaic electrode processes. arXiv preprint 2018. https://doi.org/10.48550/arXiv.1809.02930
E. L. J. Coenen and L. J. J. Janssen. Voidage of bulk solution in a tall vertical gas evolving cell. Journal of applied electrochemistry, 27(10):1143-1148, 1997 https://doi.org/10.1023/A:1018411331833
COMSOL Multiphysics (R) v. 6.2
Effective diffusivity in porous materials, application id:978.https://www.comsol.nl/model/effective-diffusivity-in-porous-materials-978.Accessed: 2021
M. Doyle, T. F. Fuller, and J. Newman. Modeling of galvanostatic charge and discharge of the lithium/polymer/insertion cell. Journal of the Electrochemical society, 140(6):1526, 1993 https://doi.org/10.1149/1.2221597
N. Epstein. On tortuosity and the tortuosity factor in flow and diffusion through porous media. Chemical engineering science, 44(3):777-779, 1989 https://doi.org/10.1016/0009-2509(89)85053-5
T. F. Fuller and J. N. Harb. Electrochemical engineering. John Wiley & Sons, 2018
J. W. Haverkort. A theoretical analysis of the optimal electrode thickness and porosity .Electrochimica Acta, 295:846-860, 2019 https://doi.org/10.1016/j.electacta.2018.10.065
A. A. Kulikovsky. How important is oxygen transport in agglomerates in a pemfuel cell catalyst layer? Electrochimica Acta, 130:826-829, 2014 https://doi.org/10.1016/j.electacta.2014.03.131
A. A. Kulikovsky.Analytical modelling of fuel cells. Elsevier, 2019. https://doi.org/10.1016/B978-0-44-464222-6.00012-5
C. Lamy and P. Millet. A critical review on the definitions used to calculate the energy efficiency coefficients of water electrolysis cells working under near ambient temperature conditions. Journal of power sources, 447:227350, 2020 https://doi.org/10.1016/j.jpowsour.2019.227350
X. Liu, F. Peng, G. Lou, and Z. Wen. Liquid water transport characteristics of porous diffusion media in polymer electrolyte membrane fuel cells: A review. Journal of Power Sources, 299:85-96, 2015 https://doi.org/10.1016/j.jpowsour.2015.08.092
T. Maoka. Electrochemical reduction of oxygen on small platinum particles sup-ported on carbon in concentrated phosphoric acid-i. effects of platinum contentin the catalyst layer and operating temperature of the electrode. Electrochimica acta, 33(3):371-377, 1988 https://doi.org/10.1016/0013-4686(88)85031-X
N. Nagai, M. Takeuchi, T. Kimura, and T. Oka. Existence of optimum space between electrodes on hydrogen production by water electrolysis. Internationaljournal of hydrogen energy, 28(1):35-41, 2003 https://doi.org/10.1016/S0360-3199(02)00027-7
R.Parsons. General equations for the kinetics of electrode processes. Transactions of the Faraday Society, 47:1332-1344, 1951 https://doi.org/10.1039/tf9514701332
M. L. Perry, J. Newman, and E. J. Cairns. Mass transport in gas-diffusion electrodes: a diagnostic tool for fuel-cell cathodes. Journal of the Electrochemical Society, 145(1):5, 1998 https://doi.org/10.1149/1.1838202
D. G. L. Plett. ECE4710/5710: Modeling, Simulation, and Identification ofBattery Dynamics, 2019
T. Shinagawa, A. T. Garcia-Esparza, and K. Takanabe. Insight on tafel slopes from a microkinetic analysis of aqueous electrocatalysis for energy conversion. Scientific reports, 5(1):13801, 2015 https://doi.org/10.1038/srep13801
P.J. Sides. Phenomena and effects of electrolytic gas evolution. In Modern aspectsof electrochemistry, pages 303-354. Springer, 1986 https://doi.org/10.1007/978-1-4613-1791-3_6
K. Stephan.Heat transfer in condensation and boiling. Springer, 1992 https://doi.org/10.1007/978-3-642-52457-8
M. E. Suss, K. Conforti, L. Gilson, C. R. Buie, and M. Z. Bazant. Membraneless flow battery leveraging flow-through heterogeneous porous media for improved power density and reduced crossover. RSC advances, 6(102):100209-100213, 2016 https://doi.org/10.1039/C6RA22608F
W. Tiedemann and J. Newman. Maximum effective capacity in an ohmically limited porous electrode. Journal of The Electrochemical Society, 122(11):1482,1975 https://doi.org/10.1149/1.2134046
B. Tjaden, S. J. Cooper, D. J. L. Brett, D. Kramer, and P. R. Shearing. On the origin and application of the bruggeman correlation for analysing transport phenomena in electrochemical systems. Current Opinion in Chemical Engineering,12:44-51, 2016 https://doi.org/10.1016/j.coche.2016.02.006
C. W. Tobias. Effect of gas evolution on current distribution and ohmic resistancein electrolyzers. Journal of the Electrochemical society, 106(9):833, 1959 https://doi.org/10.1149/1.2427506
B. Vijayaraghavan, D. R. Ely, Y.-M. Chiang, R. García-García, and R. E. García. An analytical method to determine tortuosity in rechargeable battery electrodes. Journal of The Electrochemical Society, 159(5):A548, 2012 https://doi.org/10.1149/2.jes113224
F. Y. Zhang, X. G. Yang, and C. Y. Wang. Liquid water removal from a polymerelectrolyte fuel cell. Journal of the Electrochemical Society, 153(2):A225, 2005. https://doi.org/10.1149/1.2138675
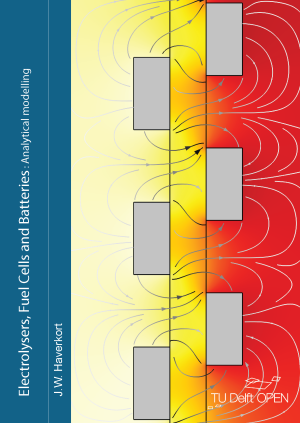